The 12 new advent windows about Acoustics I created this year
This year’s advent was a mix of old and new windows, below are the ones that we’re new for this year. At the bottom of the page you’ll find links to the old ones. Which advent worked for you? What should I try for 2021, please comment below.
1. Decibel scale
Day 1 of the 2020 #AcousticAdvent #YearOfSound ๐ A #Christmas themed dB scale is today’s offering. But what other sound levels should be on the scale?
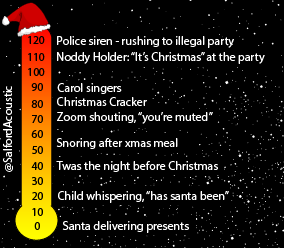
2. Father Christmas tests his #HoHoHo in Thurgoland
#SoundOn ๐ Father Christmas tests the warbling reverb in Thurgoland railway tunnel ๐ Youtube Dave Sowden shouted #Ho to test the acoustics, I turned it into #HoHoHo. Warble comes from repetitive sound paths. #AcousticAdvent #BilliardMathematics.
3. Tuba lips
A brass band playing Christmas Carols. This is what happens to the lips of the brass players ๐ฎ #HighSpeedVid The lips vibrate open and closed to break up the airflow and make sound. #AcousticAdvent #YearOfSound ๐ต๐บ
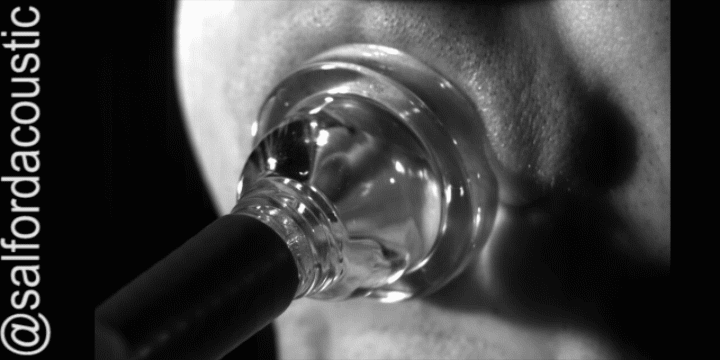
4. Bad cracker jokes
๐ or ๐ Acoustic Christmas cracker jokes #AcousticAdvent #YearOfSound
Q: How do you organize a library of sound?
A: By using the Dewey Decibel system.
My favourite niche joke, thanks @Daniel_Goodhand
Why shouldn’t you take the Fourier transform of a square pulse while on a boat? Because it will sinc.
5. Zoom Bingo
Tired of poor quality sound on video calls? Play Zoom Audio Bingo so your friends/family/colleagues learn how to improve the sound ๐ #AcousticAdvent ๐๐๐ง๐ค #YearOfSound

6. Duck on lady #slowmo
Sound created by impact #AccelerationNoise #FragmentsVibrating #HighSpeedCamera We short these to use in curriculum resources for physics. #AcousticAdvent ๐ฆ๐ฅ
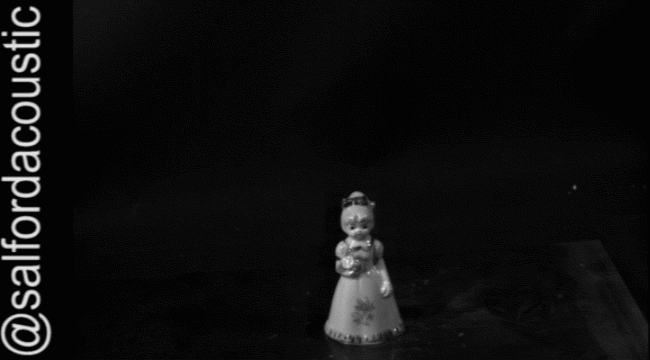
7. Carols and hearing loss
What does a carol sound like with a hearing loss and a hearing aid? #SoundOn #AcousticAdvent @epsrc @clarityprojuk is launching machine learning challenges to improve hearing aids in Jan 2021. Take part: http://claritychallenge.org/ ๐ถ๐ฆป
8. Ice shelf vibrations
Winds blowing across Antarcticaโs snow dunes cause the ice slab to vibrate. The drumroll of seismic sounds is audible because it has been pitch shifted by x1200. https://youtu.be/w56RxaX9THY Vidwo @Tenacious_She @theAGU #CCYoutube #AcousticAdvent ๐ง๐#SoundOn + ๐ง
9. Mysterious melody
A mysterious melody auditory illusion for Christmas. #SoundOn #AcousticAdvent #YannyLaurel2020 Shows how top-down auditory processing can affect what you perceive @DianaDeutsch ๐ต ๐ ๐ง
10. Analysis of Christmas music lyrics
What words should you use to get a Christmas hit? Analysis of lyrics of top 50 songs (top 50 from Timeout). #AcousticAdvent ๐งโ๐ค๐

11. Stonehenge
It’s the winter solstice so here’s a pic of Stonehenge, which was prob. orientated to where the sun rose on this day. Not the real Stonehenge, but our 1:12 scale acoustic model in Salfordโs semi-anechoic chamber #AcousticAdvent ๐ ๐ผResults from our expts.
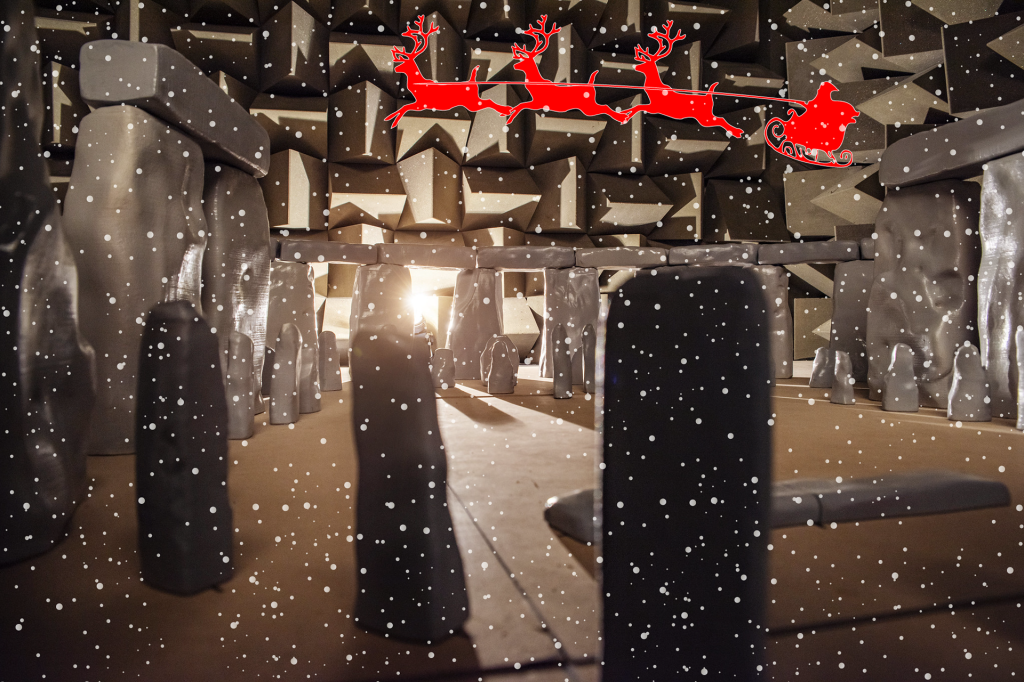
12. Foley Artist
How a foley artist creates the sound of skiing โท๏ธ for movies. I once visited the Foley stage at @warnerbros, fascinating. #AcousticAdvent
Links to old Acoustic Advent from 2019
What worked for you? What should I try in 2021, please comment below.